Project Description
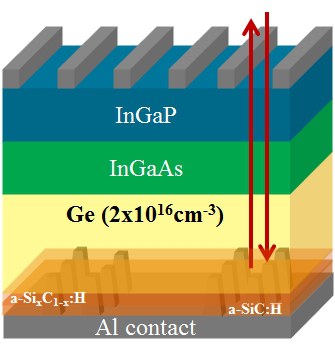
Solar cells are the preferred method for powering today’s satellites. The cell efficiency determines the available power and is hence of exceptional importance for any spacecraft equipment or system. Besides the efficiency that is directly linked to the solar array power (W/m2), solar cells define also further Key Performance Indicators such as specific mass (kg/m2) and manufacturing costs (EUR/W). The SiLaSpaCe project will address these needs by developing next generation, high performance, GaInP/GaInAs/Ge multi-junction space solar cells with reduced weight, high radiation stability and increased efficiency. This ambitious goal will be achieved by the introduction (spinning-in) of Si photovoltaic technologies which are absolutely new for space applications. These measures will allow the introduction of thinner Ge wafers and metamorphic top structures leading to increased efficiency and to reduced weight. This innovation will bring GaInP/GaInAs/Ge multi-junction space solar cells significantly beyond state-of the art lattice-matched GaInP/GaInAs/Ge triple-junction solar cells with a Beginning-of-Life (BOL) efficiency of 30%. In the SiLaSpaCe project, GaInP/GaInAs/Ge multi-junction space solar cells shall reach a BOL efficiency of 33%. The SiLaSpaCe developments will enhance new and key enabling technologies (KET) like energy production, materials and structures as well as additive layer manufacturing techniques. Finally, the project aims to attract terrestrial technologies to space systems and mobilizes new incorporations of non-space actors into the space landscape.
Work Package Descriptions
Work Package 1 Surface passivation of Germanium wafers
Pulling a crystal with a doping gradient
We develop pulling conditions that allow growing a crystal with a doping gradient covering the appropriate range of interest for this project. This crystal provides wafers to test the extremes in doping range and see its impact on passivation and IR absorption.
Deliver wafers with different thicknesses and surface roughness
We prepare wafers with three different thicknesses that are used to extract the minority carrier bulk lifetime and the surface recombination velocity from the lifetime measurements. This allows us to quantify the quality of the surface passivation. At the same time, wafers with different surface roughness are prepared (double side polished vs double side ground vs double side etched) in order to quantify the influence of the surface roughness on the surface passivation quality. Once all the different parameters have been investigated, wafers with the final optimal specifications will be made for device fabrication.
Optimise etching after grinding
After grinding a damaged layer with a certain thickness is left behind. The thickness of this layer depends on the mesh size of the grinding wheel that is used. This layer impacts the lifetime. Etching this damaged layer away while investigating the impact on the lifetime tells us how much etching is necessary for an optimal process when thinning down devices.
Wafer passivation
In a plasma enhanced chemical vapour deposition (PECVD) tool from Roth&Rau we develop a H-plasma cleaning procedure to remove all oxide residuals on the Ge surface. In an in-situ process a SixC1 x:H passivation layer is deposited. As an alternative wet chemical treatments is tested and compared to the two step plasma process. Once the pre-treatment is defined, the process parameters for the SixC1 x:H passivation layer together with potential post-annealing treatments is fine-tuned.
Lifetime measurements
All passivated wafers with different dopings, thickness and roughness are measured with the microwave photoconductance decay (µPCD) setup. The lifetime maps quantify the uniformity of the minority carrier lifetime values. The measurement of wafers with different thickness is used to extract the surface recombination velocity for different process settings, for different doping levels and for various backside roughness values. After lifetime measurements these wafers serve for radiation tests (WP2) or optical tests (WP3).
Work Package 2 Accelerated aging and characterization
Electron irradiation aging of Ge “lifetime samples”
The performance of electron accelerated ageing at the SIRIUS irradiation facility at different fluence rates is investigated. For that purpose Ge wafers with a lower p doping concentration ranging from 1x1016 cm-3 to 7x1016 cm-3 (instead of 5x1017 cm-3) and with an a-SixC1-x:H passivation layer are provided from WP1. At these low levels of p doping, the defect content produced during electron irradiation is equivalent and has therefore a strong influence on the properties depending on the electronic nature (p or n) of the defect and its content. The fluence inside the p doped Ge wafer is the parameter controlling the electrical properties of the Ge layer junction and the lifetime of minority carriers measured by µPCD. All irradiated samples are subjected to µPCD measurements in order to analyze the changes in the free carriers lifetime as a function of the electron fluence rate. With this approach we obtain the BOL and EOL electrical parameters of the different bottom Ge/a-SixC1-x:H junctions.
Back side Proton irradiation of new solar cell back structure
The goal of the SiLaSpaCe project is to develop a new MJ solar cell back structure with an a-SiC based layer stack acting as a surface passivation (a-SixC1-x:H) of the Ge bottom cell as well as a mirror (a-SiC:H) for long wavelength light (> 1800 nm). We study the influence of irradiation on the optical as well as on the electrical performance of this SiC layer stack. As the optical SiC layers is close to stoichiometric we expect a shielding effect for the Si rich passivation layer. For electron irradiation, the number of defects produced is low (few ppm) and does not change both the structure and the surface state of the a-SiC layers. By contrary, protons of 1 MeV have a penetration depth of around 12 µm and one proton produces around 103 defects with a heterogeneous distribution of energy deposit along his course. An enhanced proton irradiation effect could therefore be observed on the reflectivity and passivation properties depending on the thickness of the a-SiC layer stack. We probe the possible changes under proton irradiation at the interface between the Ge and the a-SixC1-x:H layer. For that purpose, we perform proton irradiations at different energies using the JANNUS Orsay irradiation facility. The choice of the proton beam energies is effected using SRIM code (http://www.srim.org/) (material data from ISE) in order to perform back side proton irradiation with protons stopping in the optical a-SiC layer, around the Ge/a-SixC1-x:H layer interface and in the Ge layer. The reflectivity property measurements after the different proton irradiations and the µPCD measurements in order to analyse the changes in the free carriers lifetime is performed in WP3.
Electron irradiation aging of MJ space solar cells with new back side structure
The last task in the WP2 is the accelerated electron ageing of MJ solar cells with newly developed back side structures developed along the project and realized in WP4 (AZUR). For that task, room temperature irradiation using 1 and 2 MeV electrons are performed for different fluence rates up to 1016 e.cm-2 on several 4 cm2 square cell samples from WP4. The data are used to improve the results statistics and determine error margins in BOL and EOL parameters for the new MJ solar cell back structure.
Work Package 3 Ge back side processing (passivation, mirror and contacting)
Development of SiC layer stack for surface passivation and enhanced reflection
In a plasma enhanced chemical vapour deposition (PECVD) tool from Roth&Rau we combine the best SixC1‑x:H passivation layers which are developed in WP1 with Si and C based layer stacks with much lower refractive index (higher C content). This layer stack, together with the metallization developed inside this WP, lead to almost total reflection of light penetrating the whole solar cell (lambda >1800 nm) at the back side, therefore significantly reducing solar cell temperature. Another important role of the second SiC layer is acting as a dopant source for boron which is important for further laser processing. Reference systems with a passivation and an anti-reflection coating on the front side are fabricated to measure effective lifetimes of minority carriers in the Ge bulk and enable reflection and transmission measurements.
Laser ablation of SiC and dopant in diffusion
Using a solid state IR/VIS laser the SiC layer stack is removed locally on areas of around 50-90 µm diameter (step width around 1-3 mm in all directions). During the ablation process, boron from the SiC layer diffused locally into the Ge wafer and form a p++ region under the contact areas. This p++ region enables a metal/Ge contact with low recombination velocities for minority carriers. Using cross section micrographs and electron beam induced current (EBIC) measurements, the structural and electrical performance of the contact areas is characterized in detail. Finally, reference systems with a passivated front side are fabricated to measure recombination velocities on the contact areas and effective lifetimes of minority carriers in the Ge bulk. The balance between sufficient doping concentration and minimized damage in the Ge wafer is another main issue.
Metallization of Ge back side
Several materials for metallization are applied. Evaporation of Ag/Au layer stacks is used as these two materials are standardly used for space applications. As an alternative Al/Ag or Al/Ti/Pd/Ag stacks is applied as these more cost-effective stacks of metals have shown also very promising results so far. For laser fired contact formation, only Al/Ag stacks are used as the high Al content is of utmost importance for the local p+ contact interface. Especially for this approach, subsequent annealing of the samples up to < 300°C for < 30 min is done to enable a low contact resistivity.
Laser fired contact formation
Using a solid state IR laser the metal layer on top of the SiC stack is molten and penetrates into the Ge wafer. Al forms a locally highly doped (p+) region under the contact area of Al and Ge. This p+ region enables a metal/Ge contact which enables low minority carrier recombination velocities. Using cross section micrographs (SEM) and electron beam induced current (EBIC) measurements, the structural and electrical performance of the contact areas is characterized in detail. Finally, reference systems with a passivated front side are fabricated to measure recombination velocities on the contact areas and effective lifetimes of minority carriers in the Ge bulk. The major issue is to balance local heat injection such as to form an excellent electrical contact without introducing too much stress into the Ge wafer.
Work Package 4 Solar cell simulation, fabrication and characterization
Simulation of a multi-junction solar cell structure with improved Ge sub-cell (ISE)
A theoretical estimation of the BOL and EOL performance of multi-junction solar cells with improved Ge sub-cell are performed. As an input, experimental data on the achieved performance of Ge sub-cell is used. The effect of IR reflector on the cell temperature and thus performance is taken into account. Based on the simulation results, the structure of upper sub-cells is optimized to meet the requirement on a perfect current matching.
Development of a manufacturing route
All R&D activities at AZUR are performed with the production equipment also used for regular products. Thus, the introduction of new processes will require in-depth tests on compatibility of these processes and new materials to the established production environment. Individual process steps (photolithography, metallization, contact annealing) are optimized.
Realization of a solar cell demonstrator
This activity is dedicated to the realization of a metamorphic solar cell with improved Ge sub-cell. The process is split between AZUR and ISE. ISE performs PECVD and LCF/PassDop, AZUR all other processes. For a part of cells, standard AZUR contact metallization are applied to the back side though the openings in the passivation. Manufactured solar cells are characterized at reference conditions and at BOL and EOL. The verification against project targets is done.
Technology evaluation
The results of realized manufactured routes is evaluated and quantified in terms of product performance, process compatibility and costs. The technology industrialization road map is prepared.